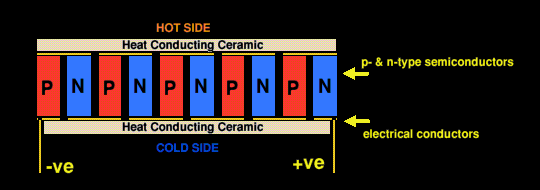
A thermo-electric cell.
COMPACT NUCLEAR POWER SOURCES
Compact nuclear power sources usually rely on the self-generated heat that highly radioactive nuclides produce when they undergo nuclear decay. A thermopile is often used to harness the heat given off by the decay. The thermopile generates an electrical current. Thermopiles consist of a 'battery' of two different metals stacked one upon the other in an alternate fashion. In effect it is a stack of thermocouples connected in series, where alternate junctions are exposed to a heat source. The other set of junctions is kept as cold as possible. This generates a voltage by the Seebeck effect. If the circuit is completed, an electrical current will flow. The greater the temperature difference between the junctions, the greater the electrical power generated. A temperarure differential of 400 degrees Kelvin can usually be attained. By this means a radio-nuclide can power the electronics within a spacecraft. They are more properly called radioactive thermoelectric generators (RTGs). The metals used can be n- and p-doped semiconductors. RTGs are also used as the sole power sources for remote lighthouses and navigation bouys. Russia deploys a great many such devices, few are protected against theft. Electrical outputs of RTGs vary from just a few Watts to a few kiloWatts; some are the size of cars, others the size of vacuum flasks.
In order for the energy from radio-nuclides to be harnessed effectively, the emitted energetic particles (alpha, beta and gamma rays) must be stopped, whereby their energy is converted into heat. Not all the energy unleashed by the isotope can be converted into heat in this way. Some particles take part in further nuclear reactions, and some are thus re-absorbed with a part of their energy being converted into binding energy again, which is not liberated as heat. Some other particles escape altogether. The Watts per gram figures quoted is the total power produced by the nuclide (including the possible decay of all it's daughter nuclei) in decaying. Not all this power can be converted to heat, and even less subsequently into electrical power.

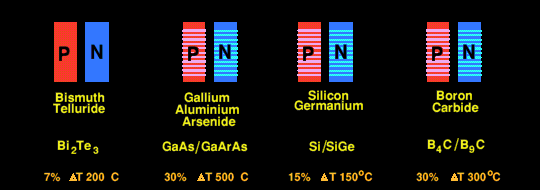
Efficiencies of Various Semiconductors used in Thermo-Electric cells.
The efficiency of Bismuth Telluride (Bi2Te3) thermoe-electric cells themselves is about 7% at a temperature difference of 200 Celsius. There are new experimental high efficiency quantum well thermo-electric cells using, variously, n- and p- doped thin alternate layers of SiGe and Si; or thin alternate layers of two forms of boron carbide (B4C and B9C which can reach efficiencies of 25% at temperature differences of 250 Celsius.
Thermo-electric cells will also work in reverse; if a current is passed through the device, then one side will get hot whilst the other side will get cold, this being a Peltier Cooler. Which side gets hot and which one cold depends upon the direction of the electrical current. Peltier Coolers are used to cool CCD sensors in astronomical telescopes, in order to reduce the thermal noise they generate. Others transport the heat produced by microprocessor chips to heatsinks more effectively than could be done otherwise.
A solid-state device that reaches sub-cryogenic temperatures has now been constructed. See Cryogenic Peltier Cooler
The overall conversion efficiency, nuclear energy to electrical power, is likely to be quite low, probably between 1% and 10%, depending upon construction.


TYPICAL HEAT SOURCES FOR THE THERMOPILES

Simulation [Pop Out Page]
Promethium-145, with a half-life of 2.5 years, is the longest lived isotope of promethium, and has a specific activity of 940 Curie per gram. It is a soft beta emitter, and although no gamma rays are emitted, X-radiation can be produced when beta particles impinge on elements with a high atomic number, and great care must be taken in handling it. Promethium salts luminesce in the dark with a pale blue or greenish glow, due to their high radioactivity. Promethium-145 is used as a beta source for thickness gauges and for luminescent signs where the beta rays strike a phosphor producing light, that light can also generate electricity in a photocell giving a nuclear battery a useful life of 5 years. Promethium could be used as a portable X-ray unit, and as a heat source in thermopiles to provide auxiliary electrical power for space probes and satellites.

Simulation [Pop Out Page]
Curium-242, releases about 122 watts per gram, compared to just half a watt for plutonium-238, suggesting uses as an isotopic power source, but it has a short halflife of only 168 days. Small quantities of curium are also produced incidentally in nuclear explosions, and occur in nuclear fallout.

Simulation [Pop Out Page]
Polonium-210 is now made in milligram quantities by bombarding bismuth-209 with neutrons creating bismuth-210, which decays with a halflife of 5 days into polonium-210. Polonium-210 is an alpha emitter, with a halflife of 138 days and emits 5000 times more alpha rays than does the same mass of radium. Producing 144 watts per gram, a half a gram can reach 500 Celsius, making it useful as the energy source for thermo-electric generators in spacecraft. Polonium-210 emits a blue glow in air, caused by air ionization. Polonium-210 is exceedingly hazardous even in microgram quantities due to the complete absorption of its huge flux of alpha particles by human tissue. The maximum allowable ingestion of polonium-210 is just 7 picograms.

Simulation [Pop Out Page]
Plutonium-238. NASA has used plutonium-238 as the radioactive heat source. Plutonium-238 has a halflife of 87.7 years, and decays primarily by alpha decay, with a very small proportion decaying via spontaneous fission. Plutonium-238 will only produce 0.567 watts per gram, but it is available in plentiful supply from the processing of fuel from nuclear power stations. NASA uses it in the form of plutonium dioxide because that is more manageable and safer in the event of a crash to Earth. Containment of radiation is paramount aboard a spacecraft. Additional physical protection is provided in the event of a crash or re-entry of Earths atmosphere to prevent escape of the radio-nuclide.

Simulation [Pop Out Page]
Strontium-90. Both Strontium-90 and plutonium-238 have been used in Russian radioactive thermoelectric generators. Strontium-90 is another product of nuclear fission within nuclear power stations, and is readily extracted from the waste during reprocessing. Strontium-90 is a beta emitter with a half-life of 28.8 years and liberates a useful 2.25 Watts per gram.

Uranium-233. A 100kg of U-233 will provide 100kW of electrical power from fission in the SAFE (Safe Affordable Fission Engine) that the Los Alamos National Laboratory is working on. It uses 200 rods packed with uranium-233 oxide in a hexagonal array surrounded by 6 larger cylinders containing a neutron reflector on one side, and a neutron absorber on the other. To switch the reactor on, the 6 cylinders are rotated half a turn so that the neutron reflectors reflect escaping neutrons back into the core, triggering fission. To turn the power down the cylinders expose their neutron absorbing side to the core. The heat produced from the core is removed by a gas or liquid that is then used to drive the cylinder of a Sterling engine and thence a dynamo to generate the electrical power. The efficiency of a Sterling engine is 23%, whilst that of some thermo-electric piles only 6%. But the moving parts must be reliable, there are no moving parts in a thermo-electric generator. Uranium-233 is only mildly radioactive, so should present little hazard if things went wrong on launch. Dangerously radioactive isotopes of fission only accumulate when the reactor is switched on.
POWER SOURCES USING OTHER PRINCIPLES
It may be possible to construct very small, but radiologically safe, personal power generators using beta radiation to directly generate electricity within a p-n junction. The beta particles (electrons) given off by a beta decaying isotope, such as the radioactive hydrogen isotope, tritium, can be trapped by a semi-conducting p-n junction where a voltage will be generated across the junction. The tritium gas would be contained as the solid compound, titanium tritide. The absorbtion of beta particles can be increased 400-fold (and hence also the conversion efficiency) by putting the titanium tritride at the bottom of cylindrical holes with a 100:1 depth to width ratio in the n-type semi-conductor gallium phosphide, where the walls of the holes are doped to create a p-type semi-conductor and hence a p-n junction over their whole surface. The holes, of which there would be would be thousands, would be created by deep etching. Using deep shafts in which to put the beta emitter ensures that only small amounts of the radioactive isotope are needed to produce the electrical power.